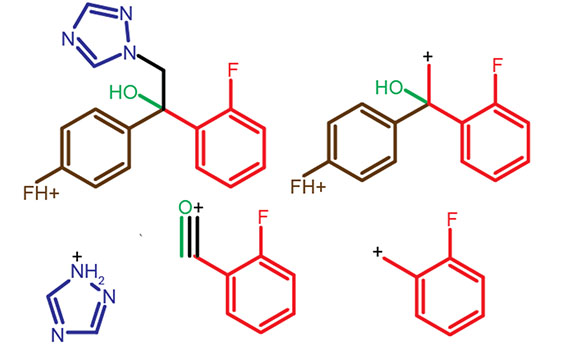
Fungicide biotransformation
- Goal: To better understand and quantify the plant contribution to fungicide longevity and efficacy through breakdown and biotransformation using tandem mass spectrometry and chemistry.
- Collaborators: Organic chemistry with Hendra Gunosewoyo (Curtin), computational chemistry Amir Karton (UNE), fungicide resistance Fran Lopez-Ruiz (Curtin), and sharing MS/MS expertise with Cynthia Joll (Curtin).
- Funding Sources: Grains Research and Development Corporation (GRDC) funding of Phase III (2022-2027) for Centre for Crop and Disease Management at Curtin University, Project F3.3 entitled "Fungicide dynamics" in Theme D, Foundation Projects.
Diseases are a major cause of crop damage worldwide and most are caused by plant pathogenic (disease-causing) fungi. Fungicides are a class of pesticides used to protect crops by specifically inhibiting or killing a fungus causing disease.
Like human medicines, agrochemicals in plants are metabolised by cytochrome P450s and glutathione-S-transferases. Unlike human medicines, fungicides usually need to be applied before disease occurs or at the first appearance of symptoms to be effective. Little is known about the lifespan of fungicides inside plants, what each fungicide is bio-transformed into or how environmental conditions or plant species affects the rate and type of biotransformation.
In addition to finding out what plants convert fungicides into, we will make the most important fungicide metabolites to answer how each major biotransformation matters? Synthesising metabolites with labels will allow accurate quantification.
Once we know the chemical ‘fingerprint’ in mass spec for each fungicide and metabolite the types of questions we will address include e.g. (i) Do all plants metabolise fungicides the same way? (ii) How do stresses affect metabolism? (iii) What contribution do attacking pathogens make to metabolism. The ability to quantify fungicides and metabolites in plant tissues offers a series of useful end-user applications as well.

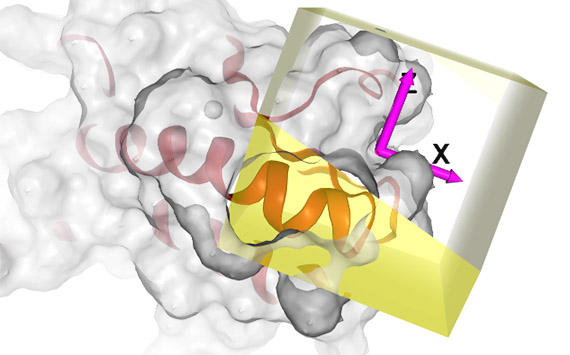
New herbicidal modes of action
- Goal: To create completely new herbicides with new modes of action
- Collaborators: Synthetic organic chemistry with Keith Stubbs (Chemistry, UWA), on DNA gyrase with Tony Maxwell (John Innes Centre), structure-based design with David Ascher (UQ) & Douglas Pires (Uni. Melb.), protein crystallography with Joel Haywood (Curtin) and Charlie Bond (UWA).
- Funding Sources: ARC LIEF LE230100156 “Integrated crystallisation facility” (Bond, Vrielink, Moggach, Mylne, Bowman, Sarkar-Tyson, Ramsay, Smith); ARC Linkage Project LP200301658 “Rethinking and revitalising herbicides to counter resistance” (Mylne, Stubbs, Lee, Williams ); ARC Discovery Project DP190101048 “Putting new herbicide targets on the table” (Mylne, Stubbs, Haywood, Maxwell); ARC DECRA DE180101445 "Structure-based investigations into new modes of action for herbicides" (Haywood); Nexgen Plants (Mylne, Stubbs & Haywood); DemAgTech (Mylne, Stubbs, Haywood & Breese).
The use of herbicides in agriculture has greatly improved crop yields through better weed management while also reducing labour and associated costs. Due to the powerful activity of these chemical molecules, many different herbicides have been developed and this has coincided with a dramatic rise in use. Consequently many different resistance mechanisms have developed (akin to antibiotic resistance) which means many frontline herbicides have waned in usefulness to the agricultural community.
Weeds are responsible for $2.5 billion in annual losses in agricultural production for Australia alone. Herbicide intensive ‘no-till’ agriculture reduces soil erosion, but all in all, this widespread use has resulted in serious herbicide resistance issues).
There is therefore an urgent need for the discovery of new herbicides with new modes of action. There are at present about 24 modes of action and resistance has evolved against almost all of them. Research has intensified recently and a couple of herbicides (aclonifen, cinmethylin) have been rediscovered with new modes of action and the truly novel tetflupyrolimet is nearing release, but apart from these cases, all ‘new’ releases for the last 30 years have been reformulations of compounds already in use.
Our lab has found many new plant target proteins that are ‘druggable’ modes of action. Making these potential targets in E. coli and moving them to biochemical assays and obtaining 3D structural information opens a range of possibilities.

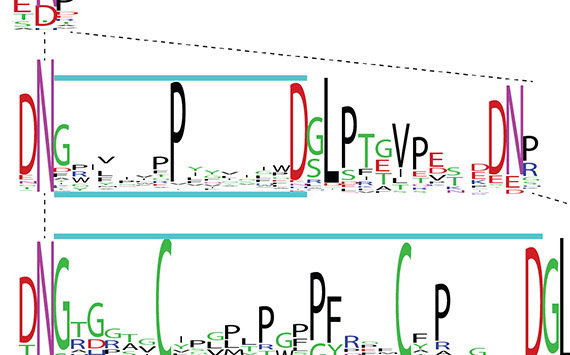
Interstitial and cyclic peptides
- Goal: To discover small and often bioactive peptides and their biosynthetic origins using de novo transcriptomics, mass spectrometry and bioinformatics.
- Collaborators: RNA-seq with Jim Whelan & Oliver Berkowitz (Agribio, La Trobe), NMR with K. Johan Rosengren (UQ), bioinformatics with Pavel Pevzner (UCSD, USA), Phylogenetics with Ed Schilling (UTK, USA), Jose L. Panero (U. Texas at Austin, USA) and Hanno Schaefer (TUM, Germany).
- Funding Sources: ARC Discovery Project DP190102058 “Buried treasure: bioactive plant seed proteins evolving inside hosts” (Mylne, Rosengren, van der Hoorn, Hara-Nishimura), ARC Discovery Project DP130101191 “A new and rapidly evolving class of seed peptides” (Mylne, Schilling, Panero).
Cyclic peptides are ultra-stable with many activities claimed for them. In plants they get cut from within precursor proteins and some have taken an evolutionary shortcut by appearing from within what then becomes a host protein - hence 'interstitial' peptides. The genetic events that created them are diverse ranging from millions to tens of millions of years old.
The huge and diverse peptide families have activities like blocking enzymes while others have drug potential. The ability to find these peptides is now accelerated by a tailored algorithm called CycloNovo, which coupled to RNA-seq and de novo transcriptomics is revealing a huge variety of natural products with potential benefits to biomedicine, plant biology and biotechnology.
We frequently extract RNA from plants known or suspected of having cyclic peptides or peptides for which no gene sequence information is known. The RNA-seq data is used to assemble transcriptomes which will reveal the gene encoding the peptides. Mass spectrometry is used to confirm the predictions and accelerate discovery of related peptides.
The main peptide classes our lab have discovered are the PDPs (single S-S bond) and PLPs (homodetic cyclic peptides, aka orbitides), which are interstitial - buried in a latent region of precursors for seed storage albumin. Another class, the VBPs, are helical hairpins buried in provicilin. We have begun exploring the origins of other cyclic peptides, like the PLPs but processed by different proteases e.g. annomuricatins and evolidine-likes.

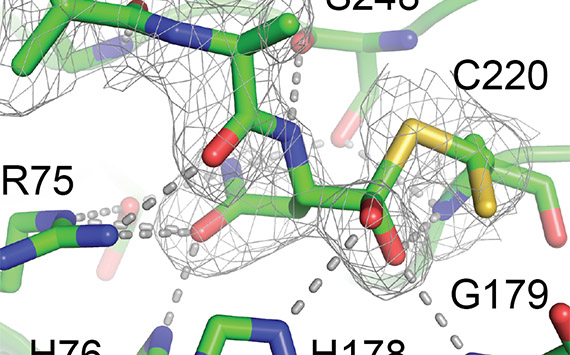
Transpeptidation by endoproteases
- Goal: To understand why and how some proteases, namely AEP, has a preoponderance for making peptide bonds as well as beaking them.
- Collaborators: Structural enzymology with Mikael Elias (University of Minnesota, USA), protein crystallography with Joel Haywood and Charlie Bond (UWA, School of Molecular Sciences).
- Funding Sources: ARC Discovery Project DP160100107 “How scissors learn to glue: the catalysis of ligation by proteases” (Mylne, Tawfik), ARC Discovery Project DP120103369 “How do sunflowers make protein drugs in their seeds?” (Mylne, Rosengren, Suga).
Proteases usually cleave peptides, but under some conditions they can also ligate them. Since before the 1930s proteases have been known to be reversible by altering solvents or concentrating substrates, but several biological examples of protease-mediated ligation have also been characterized. These include formation of an Agrobacterium sex pili protein, peptide macrocycles from cyanobacteria and several from plants.
Most petinent to our work, was that 30 years ago, an intriguing post-translational modification was shown to create concanavalin A (conA), a jack bean lectin and commercially used for carbohydrate chromatography. To make conA required the N-terminal half of the gene-encoded precursor to be swapped and become the C-terminal half of mature conA. The cysteine protease, asparaginyl endopeptidase (AEP), was shown to be involved.
Work on cyclic peptides noted a conserved proto-C-terminal Asp or Asn residue, the target for AEP - several cyclic peptide biosyntheses also appeared to have converged upon AEP for cyclization, a similar transpeptidation reaction as conA but making a constrained peptide instead of a larger protein. Macrocyclization by AEP is in effect, an iso-energetic, cleavage-coupled, intramolecular transpeptidation. Sounds complicated? It's a simultaneous cut-and-glue!
Our lab was the first to make plant AEP recominantly and we solved the first structure of a catalytically active plant AEP, where we also managed to observe electron density for a tetrahedral intermediate that predicted the binding mode. We solved the structure for a popular AEP from C. ternatea dubbed butelase 1 and it's available at Addgene. We are still pursuing structure-based mechanistic studies of AEP enzymnology.